Photon Science
Insights into fundamental material properties
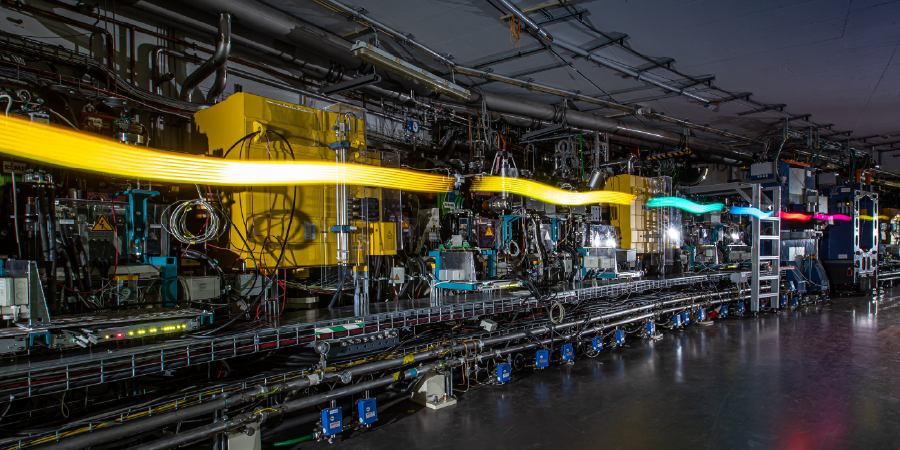
In order to optimise materials for present and future applications and to give them targeted functions, we need to understand them at the fundamental level. How, for example, does a physical effect in an atomic structure influence the behaviour of a material? How should the electrons be arranged in the atom for the material to behave as desired? Our synchrotron radiation source BESSY II is a unique facility for exploring such questions. We are constantly probing the limits of experimentation and the limits of material processes. At HZB, we are fascinated by the following questions:
- How does the functionality of a material emerge and how can we control it?
- How are chemical reactions controlled at the atomic level?
- What decides the efficiency of phase transitions and switching processes in components?
- How can we identify the limiting steps in the conversion of light into energy?
- How can we improve biochemical processes and optimise them for industrial applications?
In answering these and similar questions, the arrangement of atoms and, above all, the electronic structure in molecules and materials play a decisive role, given that it is the electrons that are responsible for almost all properties of matter.
For our research, we primarily use soft X-ray radiation in the necessary quality and intensity that only synchrotron radiation sources like BESSY II can generate. Based on the interaction between matter and X-rays, we are continually developing suitable experiments, instruments and new methodological approaches.
Example: transition metal complexes.
These consist of one or more metal atoms surrounded by many possible kinds of molecules bound to them. Such complexes act as catalysts that accelerate certain chemical reactions, including conversion of sunlight into electricity. In many of these complexes, the central metal atom is a rare and thus expensive metal, such as ruthenium as used in dye solar cells. Indisputably, it would be preferable to replace these with cheaper metals such as iron.
We are studying the properties and elementary functions of these complexes in detail. We use inelastic X-ray scattering at BESSY II to measure how much light energy is absorbed by individual molecules, and what role the neighbouring molecules in the complex play. By irradiating an iron complex with short X-ray pulses, for example, we can follow systematically how it responds to being illuminated and energetically excited with light. These and other studies will reveal what is most important in transition metal complexes for converting light efficiently into electrical energy.
Example: enzymes.
These are proteins that play a vital role in living organisms. They copy DNA, keep metabolic processes going, synthesise all important components of life, and much more. Some diseases can be treated by targetedly stimulating or blocking certain enzymes in the organism using tiny active molecules. Furthermore, bacteria can be used to produce energy by having them process various kinds of substances, for example plastics like PET, making them of great interest for industrial applications.
Our researchers aim to understand the functions of these large protein molecules at the atomic level. One can then control them with smaller molecules, or targetedly modify a component so that the molecule can accomplish a specific task such as breaking down plastics. The methods we use for these investigations are X-ray crystallography and X-ray microscopy with synchrotron radiation.
Video: BESSY II - Brilliant light for a brilliant future
Explore what happens behind the walls of the BESSY II light source in Germany. This synchrotron at Helmholtz-Zentrum Berlin attracts researchers from all over the world with its exceptionally bright light.
01:15